Langmuir-Blodgett Films
1891, Agnes Pockels was writing from Germany[1] to the future physics Nobelist, Lord Rayleigh[2] in order to submit a paper to the journal Nature; she had produced a novel technique that ultimately revolutionized how we look at the phenomenon of surface tension. She observed:
“The surface tension of a strongly contaminated water surface is variable; that is, it varies with the size of the surface".
In other words, as the aqueous concentration of surface-active species was varied, so did the apparent surface tension of the water. In her experimental device, water was trapped behind a barrier so its film properties could be measured. Rayleigh was impressed with her work and he later discovered that films of certain species floating on water could be just one molecule thick.
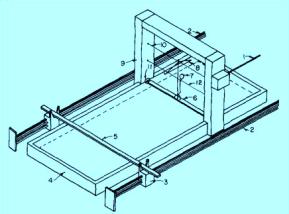
As with all good observations in the sciences, devices based on Pockel’s experiments were improved upon and were manifested as Langmuir-Blodgett (L-B) troughs (named after Irving Langmuir and Elizabeth Blodgett[3], both at a GE industrial lab in Schenectady, NY - he later received the 1932 Nobel prize in Chemistry for this other contributions to experimental chemistry and physics). A film of liquid is trapped in the trough (4 in the figure) and confined between two barriers 5 and 6. By moving barrier 5 the surface concentration of any surface-active species can be changed at will; by measuring the force necessary to compress the surface film, the properties of the film can be explored. What is meant by a surface-active species is a long molecule, one end of which is attracted to the liquid in the trough and the other is repelled from it. Laundry detergents are familiar examples; most of the molecule is oleophilic (meaning "fat-loving") but one end is hydrophilic (meaning "water-loving"). Detergent molecules thus work by compatiblizing oil and water molecules by binding both of them to the same detergent molecule.
The easiest way to understand the principles of a L-B trough is to consider a not-quite ideal gas, one in which the molecules occupy finite space. This may seem a strange analogue - a 3-dimensional gas and a film of of surface-active molecules floating on a liquid surface, but it is a useful one.
In such a gas at high pressures, i.e., when the mean free path of the molecules become comparable to the size of the gaseous molecules, their total volume cannot be neglected. The simplest model of this gaseous condition is due to the 19th century German physicist Clausius for which he wrote, where b is the volume of a mole of the gaseous molecules. Of course, as the pressure increases, the volume of gas decreases until it approaches b.
The attached graph illustrates the behavior. By measuring the hyperbolic p/V relationship, the asymptote
can be determined giving an estimate of the volume of the gaseous molecules. If you know how many molecules were present (i.e., the concentration) you also know the volume of each molecule.
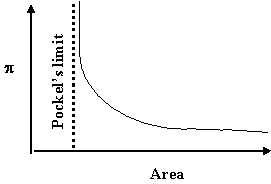
The L-B trough works on similar principles. As the surface film is compressed, the surface pressure p (i.e., the force/width of the confining barrier) increases with increasing surface molecular concentration reaching a monomolecular layer that corresponds to Pockel’s limit. At this point the surface-active species are shoulder-to-shoulder (as it were) and one can calculate their geometric footprint in this configuration. The schematics[4] below show typical shapes of p-area curves and a corresponding schematic interpretation of the geometry of the molecules of the surface-active films. Knowing the asymptotic area and the number of molecules present gives a measure of the cross section of that molecule (in that shoulder-to-shoulder configuration).
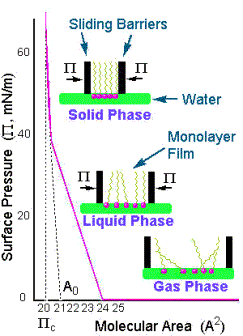
The ability to orientate molecules on a liquid surface has proven to be a very powerful self-assembly technique and has been used for amazingly diverse purposes from drug manufacture, investigations of semiconductors, manufacture of membranes for oxygen-enhancement, and for a simulation of the human tongue’s taste ability - just to name a few. A simple search on “Scirus” (the scientific version of “Google”) produces thousands of articles on L-B films and their applications[5],[6],[7],[8],[9],[10],[11],[12],[13],[14].
The reason that L-B films have proven so effective is that their molecular conformations (as described above) can be comparatively easily deduced and because their structures can be transferred to other substrates. The latter involves dipping and slowly withdrawing a substrate of interest through the film in the trough (this was Blodgett’s great contribution[15]). By ensuring that the film has an end group that will stick to the substrate it can be withdrawn with a coherent monomolecular layer; the inset diagram also shows how one can affix multiple layers to a substrate.
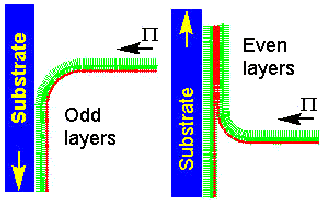
Typical molecules used as L-B films include surfactant molecules (you know them in detergents and shampoos etc.). A typical anionic surfactant is a long-chained alkyl sulphonate that has two distinct moieties (i.e., one half the chemical functionality of the molecule): an oleophilic 16-hydrocarbon alkyl chain such as the hydrocarbon chain of sodium cetyl sulphonate (C16H33SO3-Na+) and also a hydrophilic ionic sodium sulphonate end group (C16H33SO3-Na+). The long cetyl chains can be chivied at high film pressures to a practically vertical configuration on a L-B water trough; the charged sulphonate group at the other end of the molecule is attracted into the water surface. If you immerse a thin clean sheet of platinum through such a film and slowly withdraw it, the charged sulphonate end would be attracted to the platinum and the alkyl film would line up away from the metal. It would thus form a coating terminating with the –CH3 group at the end of the cetyl chain. As long ago as the 1960’s, Zisman[16] exploited this idea by substituting fluorine atoms in place of the hydrogen atoms on surfactants and obtaining both exquisitely fine control on the hydrophobicity (i.e., "water-hating") of the monomolecular fluorinated surface as well as producing the most hydrophobic surfaces ever produced. Note: the common plastic polytetrafluoroethylene (e.g., Teflon®) has an effective bifluorinated surface by exposure of its repeating units of –CF2–. It is extremely hydrophobic (as in the water repellant coating on Goretex® fabrics) but it is much less hydrophobic than the trifluorinated –CF3 surface obtained by Zisman by fully fluorinating all of the hydrogen atom sites along chain alkyl (such as the cetyl group) from beginning to end.
Virtually any outward facing coating can be applied to the appropriate surface with careful regard to its chemistry. For example, with an appropriate surfactant and liquid in the trough, if the substrate were a thin sheet of polyethylene, a hydrophobic end would be attracted to it and a charged end would face outwards. Multiple layers are a matter of aligning oleophilic-to-oleophilic or hydrophilic-to-hydrophilic end groups.
Of all ways of self-assembly, the L-B method is currently our most effective method and the one most likely to be scaled to industrial capacity ranges in the foreseeable future.
Footnotes and References
[1]. http://img.sparknotes.com/biology/cellstructure/cellmembranes/gifs/phospholipid.gif
[2]. http://www.physics.ucla.edu/~cwp/articles/pockels/pockels.html; also see: http://jchemed.chem.wisc.edu/JCEWWW/Features/eChemists/Bios/Pockels.html
[3]. http://www-gap.dcs.st-and.ac.uk/~history/Mathematicians/Rayleigh.html
[4]. K. Blodgett, J. Amer. Chem. Soc., 57, 1007, (1935)
[5]. http://www.public.iastate.edu/~miller/nmg/lbfilms.html
[9]. P.M.S. Ferreira, A.B. Timmons, M.C. Neves, et al., “Langmuir-Blodgett manipulation of capped cadmium sulfide quantum dots”, Thin Solid Films, 389 272-277, (2003)
[13]. Metzger, RM, “Monolayer rectifiers”, J. Solid State Chem., 168 (2): 696-711, (2002) [15]. K. B. Blodgett, J. Am. Chem. Soc., 57, 1007, (1935) [16]. W. A, Zisman, Adv. Chem. Ser. No. 43, (1964)