Nanocarbons
In the mid 1980’s Prof Richard Smalley and his colleagues were wondering why there appeared to be stable compounds of elemental carbon with a molecular mass of 720. This material could be observed both in deep space as well as produced in the tiniest quantities in the laboratory. In 1996 Smalley, Harold Kroto and Robert Curl received the Nobel Prize in Chemistry[1] for the discovery of “buckminister fullerene” or “bucky balls”. Their structure and properties[2] has produced much activity in the chemistry and materials sciences communities.
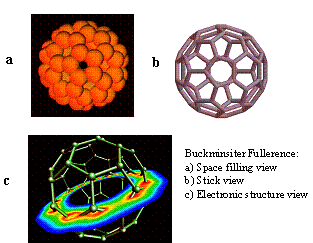
The now familiar soccer ball structure for C60 (i.e., elemental carbon with a molecular mass of 720) is shown here in several representations; it forms an open cage into which guest atoms can be located. While exciting to chemists, it may not have reached the public’s consciousness had it not been for a technologist working for a Japanese electronics company, Sumio Iijima[3], who in 1991 discovered carbon nanotubes whose structure was similar to bucky balls but with an extended length[4].
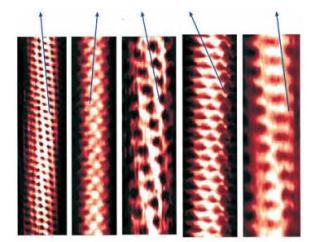
The end caps of a nanotube are one of a half bucky ball and the repeating axial hexagonal patterns are graphite structures; however, the electrical properties of nanotubes are dependent on the precise orientation[5] of the repeating hexagons. The nanotubes can be semiconductors (similar to “doped silicon” used in integrated circuits) or metal-like conductors (such as copper used as electric wiring). The inset micrographs show the orientation axes that distinguish between these electronic behaviors. These micrographs also show another important development – that of single-walled nanotubes or SWNT. Using optimum synthesis conditions one can make SWNT tubes with diameters of 1.38 ± 0.02 nm, very close to the diameter of geometrically ideal nanotubes.
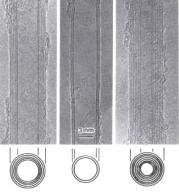
Multi-walled nanotubes are somewhat easier to make than SWNTs; under very high magnification (as per the attached inset micrographs) the multi-walled nanotubes are concentric (a good analogy is a Russian babushka doll). Whether the product is a SWNT or multiwalled is a matter of fine control. The inset diagram shows micrographs for 5, 2 and 7 MWNT respectively from left to right[2].
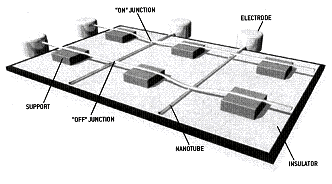
It is possible that SWNTs will find application as interconnects in nanocircuits. The attached figure is a representation[6] of such a nanocircuit. If these nano wires can indeed be made at their ideal diameter of just over 1 nm, it should be born in mind that the very best that can be realized with traditional top down technology for integrated circuits are features that are ~150 nm. In other words, this technology has the potential to shrink electronic chips by a factor of 150!
A potentially additional useful property of SWNTs is that their tensile strength is about 100 × that of steel while their density is only about 1/6 of steel. In an extrapolation [7],[8], a “Skyhook” is envisaged that will act as a space elevator suspended by SWNT cables.
The manufacture of nanocarbons (i.e., both bucky balls and tubes) is accomplished by a number of techniques. They all rely on vaporizing carbon and condensing some fraction in appropriate structures. By-and-large laser vaporization methods make relatively small amounts of nanocarbons and a specialized CVD technique is more often used for quantities in excess of a few mg. The schematic shows a generic process; high temperatures are obtained by shorting two carbon rods together causing a plasma discharge.
Such plasma can easily achieve temperatures of a few eV (recall in the section on Units that 1 eV is equivalent to ~ 11,000 K). Carbon vaporizes at about 4,500 K. Low voltages and moderately high currents[9] are needed to heat this arc. Whether one obtains bucky balls, C60, or multi-walled nanotubes or SWNTs is an “art” that is mediated by adding a catalyst such as cobalt or nickel to the carbon. Apparently small catalyst particles block the end-cap of each bucky ball hemisphere and the result is a nanotube.
In addition, an array based on a silicon crystal substrate (called a 111 face) produces a regular nanotube array[10] (in the inset picture, the scale bar is 100 nm, i.e., at the about the limit we have accepted as “nanotechnology”).
In addition to carbon nanotubes, parallel methods have been discovered to make nanotubes out of other chemicals. Thus BN, ZnO, ZnSe, ZnS, InP, GaAs, InAs, and GaN have all been made into nanotubes; the technology drive is that these nanotubes may have novel electronic properties as well as potential novel vessels for therapeutic drug delivery.
Footnotes and References
[1]. http://www.nobel.se/chemistry/laureates/1996/index.html
[2]. http://www.imbris.net/~jfromm/bucky/bucky.htm
[3]. S. Iijima, "Helical microtubules of graphitic carbon",, Nature 354, 56 (1991)
[4]. http://www.personal.rdg.ac.uk/~scsharip/tubes.htm
[5]. http://physicsweb.org/article/world/11/1/9/1
[6]. C. M. Lieber, “The incredible shrinking circuit”, Sci. Amer., p. 64., (Sept, 2001)
[8]. http://en.wikipedia.org/wiki/Space_elevator